What is fusion?
Nuclear fusion occurs when a heated plasma of hydrogen ions and electrons is magnetically moved at rapid speeds. The ions collide and fuse their nuclei, releasing energy. Fusion is the same process occurring in our sun and other stars that create heat and energy.
Fusion’s fuel — the inputs of the reaction — are abundant and relatively cheap: deuterium, a form of hydrogen with two neutrons that is found in seawater, and lithium, an element needed in an amount that is mere fractions of what is required for today’s lithium-ion batteries. To make tritium, a neutron is added to lithium (an element with three protons and three neutrons) which then decays into helium (two protons and two neutrons) and tritium (one proton and two neutrons).
Fusion occurs by colliding deuterium and tritium ions together in a reactor, which results in the production of helium ash and neutrons. Those neutrons are the energy product of fusion — they harness heat and can be used for industrial processes or converted to energy. Though they are also radioactive, they have a significantly faster decay rate compared to fission products. (Because tritium is radioactive, WHAM will run a comparable deuterium-deuterium reaction).
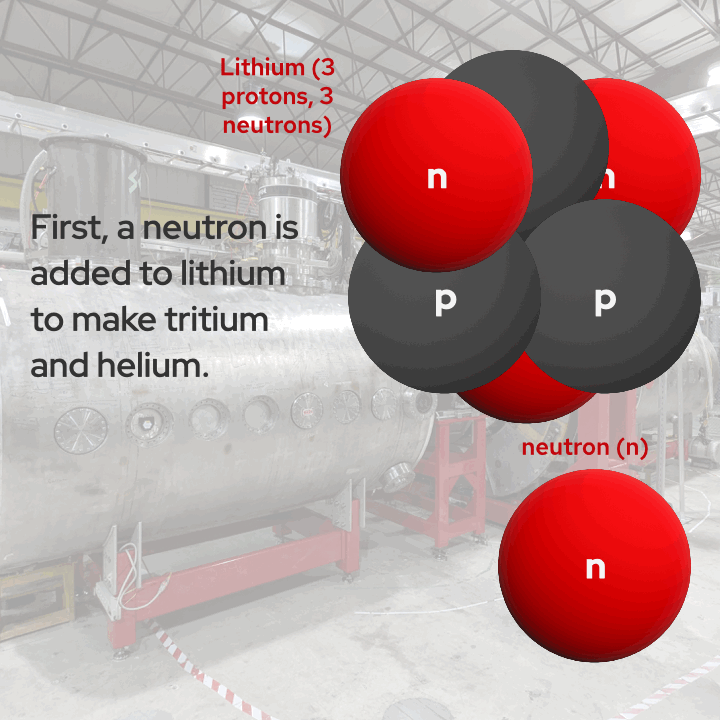
Mirror machines
Fusion devices known as mirror machines tried to replicate this process and were the basis of the U.S. fusion research program in the mid-20th century. Fusion is most efficient if the plasma is physically contained, as the particles have a better chance of colliding. Essentially a Tootsie Roll shape, the reaction center (the candy) of a magnetic mirror is a

vacuum vessel, a hollow cylinder where the plasma is contained. Because the plasma in a fusion reaction is made up of charged particles, magnets are focused inward at pinch points (the wrapper twists) outside the main reactor that function to limit plasma escape from the reactor. Lastly, they have catchment containers (the relaxed wrapper ends) as an outlet for heat and escaped plasma.
The inability to contain the plasma — and therefore an inability achieve net positive energy production — was a main factor in shutting down U.S. fusion research in the 1990s. The Department of Energy has drastically limited funding for fusion research since then (countries such as Russia and Japan have continued to make important discoveries in mirror machine research in the meantime).
Revisiting mirror machines with WHAM
In the 2010s, the breakthrough came: new, powerful, high temperature superconductor (HTS) magnets that are tens of times stronger than the previous generation and, theoretically, will be able to contain the plasma.
Superconductor magnets, which have zero resistance and create a stronger magnetic field below a certain temperature, have been around for many decades and were used in the early mirror machines, but were not strong enough to confine plasma. In the 1980s, high temperature superconductor (HTS) magnets were discovered. These magnets achieve superconductivity at relatively higher temperatures than conventional superconductor magnets — liquid nitrogen (−196 °C) vs liquid helium (−269 °C) — making them easier to work with. When HTS magnets are operated at low temperatures, such as liquid helium temperatures, the magnetic field can be several times stronger than for conventional low-temperature superconducting magnets, a breakthrough that occurred in the 2010s.
With the new magnets, the old mirror technologies, and everything learned in between by international partners, it was time to revisit mirror machines as plasma energy reactors.
In 2020, the team received a two-year, $5 million ARPA-E grant to build WHAM using HTS magnets. Two years later, ARPA-E renewed the project at $5 million for another two years. CFS is responsible for developing the magnets, and the UW–Madison team has been building the machine.